NASA/JPL-Caltech/ O. Krause (Steward Observatory)
Since the discovery of neutron stars, researchers have been using their unusual energy to study our universe. Large remnants of exploding stars, neutron stars pack more mass than the Sun into a ball about the size of San Francisco. One cup of this star material would weigh almost as much as Mount Everest.
These strange celestial objects can alert us to distant disturbances in space, teach us about the structure of matter, and reveal the secrets of how gravity and particles work in the most complex conditions in the universe.
“They are among the many open questions of space and astronomy,” says astrophysicist Vanessa Graber of the Institute of Space Sciences in Barcelona.
But in order to accurately interpret some of the signatures of neutron stars, researchers must first understand what happens inside the stars. They have their ambitions, but a direct experiment on a neutron star is not possible. So scientists need another way to test their ideas. The behavior of matter in such a dense object is so complex that even computer simulations cannot work. But researchers think they’ve found the answer: a terrestrial analog.
Although young neutron stars can have temperatures in the millions of degrees within them, by one important measure of neutron energy they are considered “cold.” Astronomers think that’s a characteristic they can use to study how neutron stars work. Instead of looking at the sky, researchers are peering into clouds of ultracold atoms created in laboratories here on Earth. And this can help them finally answer the long questions about these difficult things.
Odds in the air
The existence of neutron stars was first discovered in 1934, two years later find of neutrons alone, while astronomers Walter Baade and Fritz Zwicky he was surprised whether a celestial body made of neutrons would remain after a supernova explosion. Although they did not understand all the facts, all their views are now widely accepted.
Stars make energy by fusing the nucleus of lighter atoms into heavier atoms. But when stars break out of those light atoms, nuclear fusion ceases and there is no longer any external pressure against internal gravity. The core collapses and the outer part of the star rushes inward. When this layer reaches its dense core, it jumps and explodes outwards, creating a supernova. The core that remains behind is a neutron star.
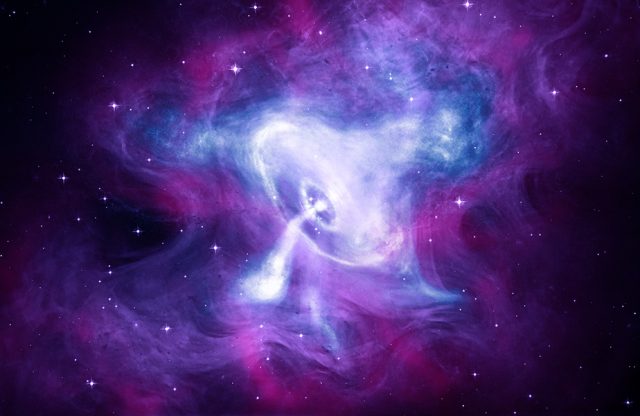
CREDIT: NASA: X-RAY: CHANDRA (CXC), OPTICAL: HUBBLE (STSCI), INFRARED: SPITZER (JPL-CALTECH)
It wasn’t until the 1960s that Zwicky and Baade’s hypothetical stars were discovered. Radio astronomer Jocelyn Bell Burnell observed a strange, high-frequency radio signal from space while working as a graduate student at Cambridge University. He was aware something that has never been seen before: A special neutron star called a pulsar, which emits sunlight over time as it rotates, like a lighthouse. (His mentor, along with the director of the observatory—but not Bell Burnell—later won a Nobel Prize for the discovery.)
Since then, thousands of neutron stars have been discovered. As some of the densest, most compressed matter in the universe, neutron stars can help us understand what happens at extreme pressures. Understanding their structure and the behavior of the neutrons they produce is of great importance to scientists.
Scientists already know that the neutrons, protons, and other particles that make up a neutron star arrange themselves differently depending on the star’s location. In some cases, they are tightly packed like water molecules in an ice cube. In some areas, they run and move like stagnant water. But exactly where the transition takes place and how the different parts do it, scientists don’t know.
A supernova star born of a nuclear fireball appears, on the face of it, to be little more than a thin cloud of ultracold particles. But they can share one important thing: They are both subject to a so-called Fermi temperature that depends on—and is calculated based on—the matter in which each system is made. A system above this temperature will behave according to the laws of classical physics; if it is down, its behavior will be governed by quantum mechanics. Some very dangerous gases and elements of neutron stars can exist below the Fermi temperature and therefore can behave similarly, says Christopher Pethick, a physicist at the Niels Bohr Institute in Copenhagen and a co-author of the previous study. an image of neutron stars in 1975 Annual Review of Nuclear Science.
Matter below the Fermi temperature can obey the laws of nature. This universe means that, even if we don’t have access to several million degrees of a neutron star, we can study its behavior by testing the ultracold gas that can be created and manipulated in laboratory reactors on Earth, he says. Astronomer James Lattimer of Stony Brook University in New York, author of a a summary of nuclear science in the 2012 Annual Review of Nuclear and Particle Science.
Of particular interest to Lattimer is a hypothetical world called the unitary gas. Air is one in that each part of its influence is infinite, meaning that it can be affected by each other no matter how far apart it is. This is impossible to achieve in reality, but clouds of superheated atoms can come close—and so do the insides of neutron stars. “It’s like fresh air, but it’s not fresh air,” Lattimer said.